The More Carbs the Better? Where's the Evidence?
While consuming carbohydrates during endurance exercise improves performance, higher doses do not consistently lead to better results.
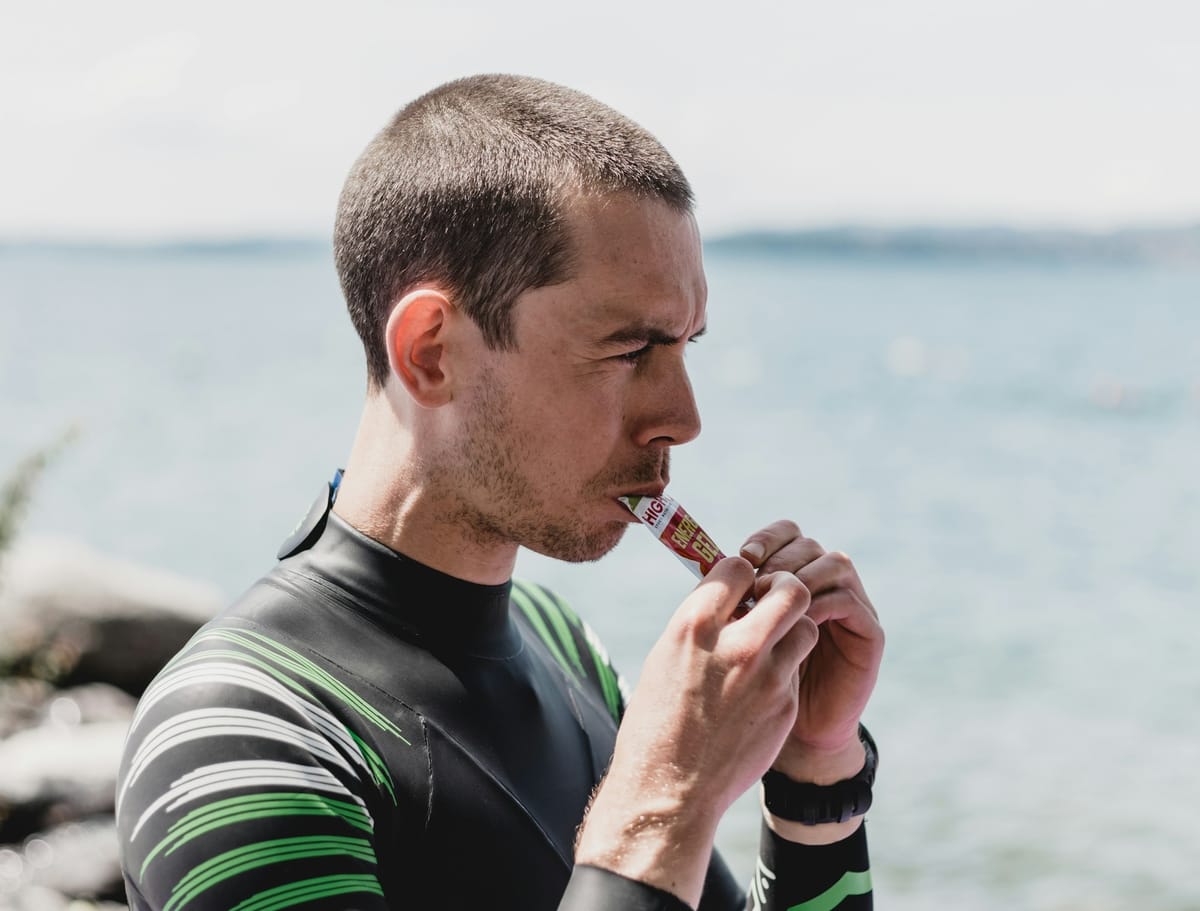
Endurance sport challenges many aspects of our physiology, not least our metabolism and our fuel stores. Our primary energy sources that broken down to power exercise are fat and carbohydrate (1). The body’s fat energy stores are vast and effectively limitless during exercise, while our carbohydrate energy stores – endogenous muscle and liver glycogen – can be depleted to low concentrations during prolonged, vigorous exercise (2–4). Therefore, a vast literature has emerged over the last ~100 years on the importance of our carbohydrate energy stores for endurance performance, and strategies to preserve them, such as through consumption of exogenous carbohydrate in sports drinks and gels. Accordingly, in endurance sport we dedicate a lot of time and effort to planning the optimal dose, type, and timing of carbohydrate ingestion before and during exercise to maximise our performance.
In this article, we are going to discuss how those of us in performance physiology have paid rather a lot of attention to the physiological effects of ingesting carbohydrates at different doses during exercise, but not quite so much attention to the effects on performance.
Performance physiologists have thought a lot about the physiology!
Early studies showed that ingesting some carbohydrates during exercise enhances endurance performance (5, 6). Physiologists proposed that these benefits were underpinned by glycogen-sparing, blood glucose-maintaining effects of the ingested carbohydrates (7–9). That is, ingesting carbohydrates during exercise reduces the burden on our limited muscle and liver glycogen stores, and helps us to maintain our blood glucose concentrations more easily. I should add here that not all studies have found that carbohydrate ingestion preserves our muscle glycogen stores (10, 11), while the evidence for liver glycogen sparing is more convincing (7–9).
Subsequently, performance physiologists turned their collective attention to optimising carbohydrate intake during exercise. This process of optimisation was not assessed against performance outcomes, but instead against what we call ‘exogenous carbohydrate oxidation rates’, which is the rate at which the carbohydrates ingested during exercise are broken down and used to support metabolism (12). The proposal has been that the greater the rate at which ingested carbohydrates are metabolised, the greater their positive effects will be.
We measure exogenous carbohydrate oxidation rates – again, the rate at which the carbohydrates in a sports drink or gel are being metabolised during exercise – using tracer isotope methodologies. This sounds complicated, and it is, so I’ll keep my explanation of it relatively brief. Carbohydrates like glucose contain carbon, and carbon typically has a molecular weight of 12. In studies of exogenous carbohydrate oxidation, participants consume carbohydrates that have been adjusted to contain carbon with a molecular weight of 13. We measure total carbohydrate oxidation by estimating the total volume of carbon dioxide (and oxygen) that the athlete expires, as when carbohydrates are broken down, oxygen is consumed and carbon dioxide is produced. We can determine what proportion of total carbohydrate oxidation is coming from exogenous sources – our sports drink modified to contain molecular weight 13 carbon – by tracking the appearance of the molecular weight 13 carbon on the breath.
The early research found that when we ingest our carbohydrates as glucose, we maximise our exogenous carbohydrate oxidation rates at ~60 grams per hour (13). However, when we ingest a mixture of glucose and fructose – two slightly different simple sugars – we can increase exogenous carbohydrate oxidation rates up to ~90 grams per hour (14–16). This increased exogenous carbohydrate oxidation likely occurs as glucose and fructose are trafficked across the gut and into the blood using different transport proteins, and the rate-limit for glucose is ~60 grams per hour. More glucose would back up in the gut. Fructose gets across using a different transporter. Accordingly, sports nutrition guidelines generally recommend carbohydrate ingestion at these rates during prolonged competitions to maximise exogenous carbohydrate oxidation rates (17, 18).
Recently, a couple of studies have looked at the metabolic effects of ingesting carbohydrates at even higher rates during exercise – up to 120 grams per hour (19, 20). These studies tell us that we don’t get any further glycogen savings when ingesting carbohydrates at these very high rates (19). All the extra exogenous carbohydrate oxidation simply displaces fat oxidation, meaning that we are burning through our glycogen stores at the same rate whether we’re ingesting carbohydrates at 90 or 120 grams per hour. In fact, research from back in 2018 suggested that increasing carbohydrate ingestion above 90 grams per hour not only fails to preserve glycogen, but may stimulate its breakdown (21, 22). This is the precisely the opposite of what we are after!
But what about carbohydrate dosage and performance?
So, if an athlete were to ask what a particular dose of carbohydrate ingestion during exercise is likely to do to exogenous carbohydrate oxidation rates, we have a hefty mass of literature to draw from. However, were they to ask what a particular carbohydrate dose is likely to do to their performance, the literature is surprisingly sparse. It’s as though performance physiology has focused on the physiology, and forgotten about performance.
The data that is out there, though, doesn’t really line up with the assumption that greater feeding and exogenous carbohydrate oxidation results in the best performance outcomes. For example, a study published in 2014 found no difference in performance outcomes when glucose was ingested at ~60 grams per hour, compared to when a 2:1 glucose-fructose blend was ingested at ~90 grams per hour (23), despite the likely greater exogenous carbohydrate oxidation in the glucose-fructose trial (14–16). The protocol used in this study was a good one, as eight trained male cyclists rode for two hours at a steady intensity, and then completed a 30-km time trial. As that adds up to ~3 hours of exercise, you’d have thought the greater carbohydrate feeding would have been helpful, but that wasn’t seen in the data. Blood glucose concentrations were similarly maintained in the trials, which suggests that both feeding rates may have exerted positive effects on performance – the participants also did a water trial in which no carbohydrate was ingested – via maintenance of blood glucose, and that the lower carbohydrate dose was ample to achieve that.
A similar study, published in 2010, had 12 cyclists ride for two hours at a very-steady intensity – 77% of their V̇O2max – and followed that with a 20-km time trial (24). They did this four times, and ingested carbohydrates at 0, 15, 30, and 60 grams per hour during the four trials. Power output was higher and performance was improved in the time trials in which carbohydrate was ingested, but there was no difference in performance when ingesting carbohydrates at 15, 30, and 60 grams per hour. One might argue that the study was a little underpowered for this many comparisons – the more comparisons you make, the less statistical power you have – but the study certainly did not provide convincing evidence that a dose-response effect of carbohydrate ingestion during exercise on performance was present.
The same group published a follow-up study in 2013, which did claim to show a dose-response relationship between carbohydrate ingestion rates and endurance performance (25). This study, which was a little unusual, had 51 cyclists complete four performance trials, which involved two hours at a steady intensity followed by a 20-km time trial. The cyclists completed the four time trials with no carbohydrate ingestion, and with three of 12 different ingestion rates between 10 and 120 grams per hour. As the cyclists didn’t complete trials with all 12 ingestion rates, the authors used a mixed-model analysis, rather than simple comparisons of performance at different carbohydrate ingestion rates.
Accordingly, it is quite tricky to make sense of the results. The overall variation in performance explained by carbohydrate ingestion rate was pretty low, as you’d expect – clearly the fitness level of the athlete matters much more than how much carbohydrate is being ingested. The modelling analysis predicted dose-response improvements in performance up to ~78 grams per hour, after which decreases in performance were predicted. Figure 1 illustrates the primary findings of this study, depicting the relationship between ingestion rates and time trial performance. The data does not convincingly support a dose-response effect, as the graph shows a relatively flat line from 30 g to 90 g of carbohydrates ingested per hour.
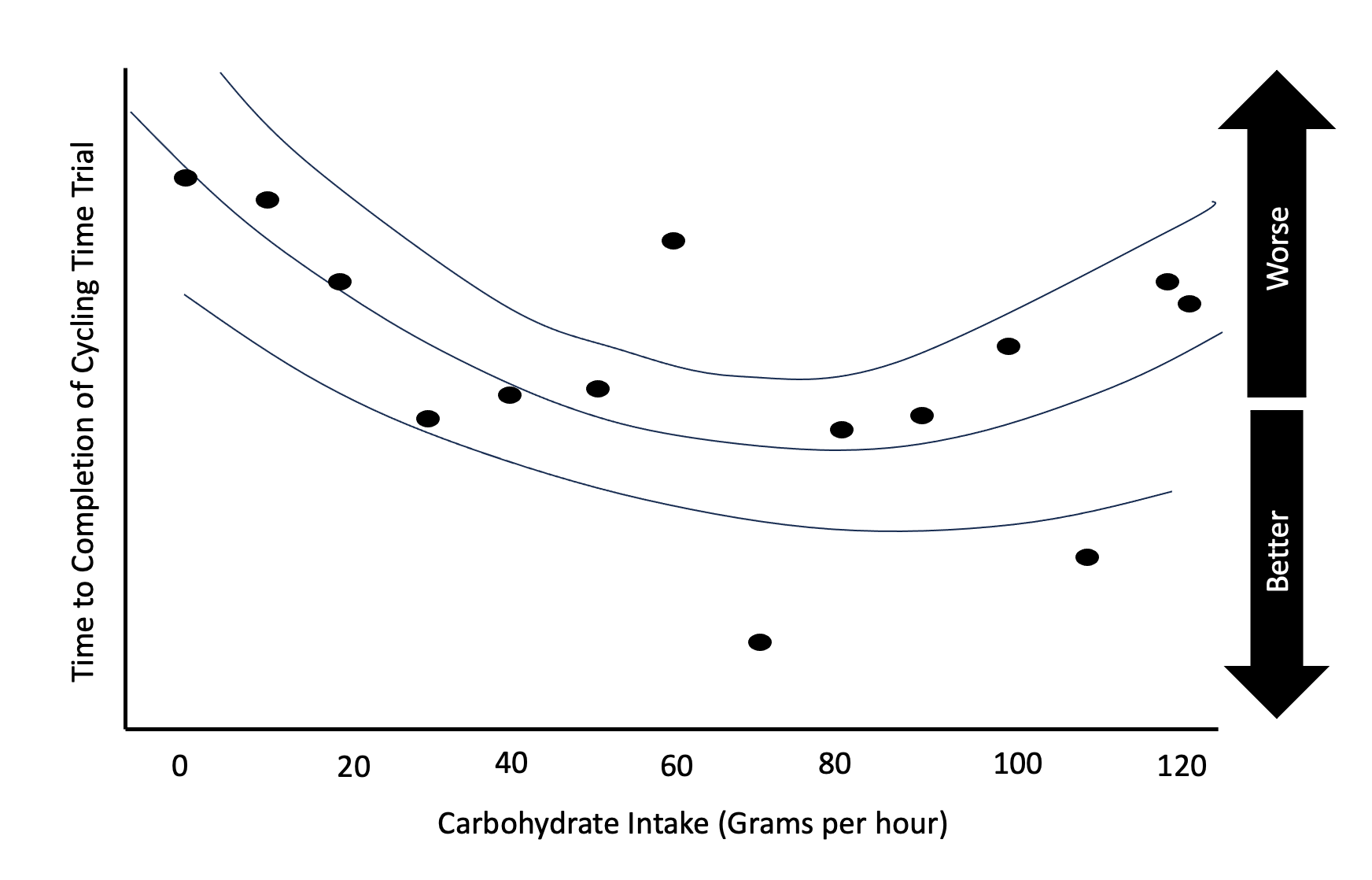
Like I said, these results are quite tricky to interpret, and do counter those I discussed previously. Since then, Andy King and colleagues published two further studies that looked at the dose-response effects of carbohydrate ingestion during exercise (21, 22). They firstly had 10 trained male cyclists ride for two hours at 77% of their V̇O2max, and then complete a 30-min time trial (22). They did this with no carbohydrate ingestion, and with carbohydrate ingestion rates of 60, 75, 90, and 112.5 grams per hour. Carbohydrate ingestion improved performance, but there wasn’t much evidence of a dose-response, with no significant differences in performance between the carbohydrate trials. There was perhaps some indication that 90 grams per hour outperformed 60 grams per hour, but this was not significant.
In their follow-up study, eleven trained males rode for three hours at 60% of their V̇O2max, and then completed a 30-min time trial (21). They did this four times – without carbohydrate ingestion, and with carbohydrate ingestion at 80, 90, and 100 grams per hour. As in their previous study, time trial performance was improved with carbohydrate ingestion, but there was no difference between the doses. Again, these two studies do cast doubt over the hypothesis that more carbohydrate is better.
As I have mentioned before, as endurance athletes, we appear to have embraced the notion that consuming more carbohydrates and enhancing tolerance equates to better performance. However, tolerance does not necessarily translate to improved performance. This belief seems to have been further intensified in recent times with the introduction of hydrogels. Indeed, in some instances, these hydrogels have demonstrated an ability to enhance tolerance (26). Yet, current research has not conclusively demonstrated that endogenous muscle and liver carbohydrate oxidation rates are reduced with this technology (26, 27). Therefore, the high carbohydrate dosages facilitated by hydrogels (>100 g per hour) are unlikely to result in subsequent improvements in performance.
Summary and practical recommendations
In summary, performance physiology has produced a stack of excellent studies reporting the effects of different carbohydrate dosing strategies on various physiological outcomes during exercise – exogenous carbohydrate oxidation rates, fat oxidation rates, and muscle glycogen breakdown. What performance physiology hasn’t produced is a stack of research reporting the performanceeffects of those different carbohydrate dosing strategies. The relatively small number of studies do not provide strong support for the assumption that more carbohydrate ingestion – via positive effects on exogenous carbohydrate oxidation rates – promotes performance. This all needs to be balanced against the risk of gastrointestinal distress, which is likely to increase as the intensity of exercise and dose of carbohydrate increases.
My take? Some is carbohydrate ingestion during competition is good, but more isn’t always better.
References
- Spriet LL. New insights into the interaction of carbohydrate and fat metabolism during exercise. Sports Medicine 44: 87–96, 2014. doi: 10.1007/s40279-014-0154-1.
- Bergström J, Hultman E. A study of the glycogen metabolism during exercise in man. Scand J Clin Lab Invest 19: 218–228, 1967.
- Bergström J, Hermansen L, Hultman E, Saltin B. Diet, muscle glycogen and physical performance. Acta Physiol Scand 71: 140–150, 1967.
- Ahlborg B, Bergström J, Ekelund L, Hultman E. Muscle glycogen and muscle electrolytes during prolonged physical exercise. Acta Physiol Scand 70: 129–142, 1967.
- McConell G, Snow RJ, Proietto J, Hargreaves M. Muscle metabolism during prolonged exercise in humans: Influence of carbohydrate availability. J Appl Physiol 87: 1083–1086, 1999. doi: 10.1152/jappl.1999.87.3.1083.
- Coggan AR, Coyle EF. Reversal of fatigue during prolonged exercise by carbohydrate infusion or ingestion [Online]. J Appl Physiol 63: 2388–2395, 1987. www.physiology.org/journal/jappl.
- Bosch AN, Dennis SC, Noakes TD. Influence of carbohydrate ingestion on fuel substrate turnover and oxidation during prolonged exercise [Online]. J Appl Physiol 76: 2364–2372, 1994. http://eutils.ncbi.nlm.nih.gov/entrez/eutils/elink.fcgi?dbfrom=pubmed&id=7928859&retmode=ref&cmd=prlinks\npapers2://publication/uuid/F479B870-C0EE-4489-A686-39EA1EA057F1.
- Jeukendrup AE, Wagenmakers AJM, Stegen JHCH, Gijsen AP, Brouns F, Saris WHM. Carbohydrate ingestion can completely suppress endogenous glucose production during exercise. Am J Physiol Endocrinol Metab 276: E672–E683, 1999.
- McConell G, Fabris S, Proietto J, Hargreaves M. Effect of carbohydrate ingestion on glucose kinetics during exercise. J Appl Physiol 77: 1537–1541, 1994.
- Coyle EF, Coggan AR, Hemmert K, Ivy JL. Muscle glycogen utilization during prolonged strenuous exercise when fed carbohydrate. J Appl Physiol 61: 165–172, 1986.
- Jeukendrup AE, Raben A, Gijsen A, Stegen JHCH, Brouns F, Saris WHM, Wagenmakers AJM. Glucose kinetics during prolonged exercise in highly trained human subjects: Effect of glucose ingestion. Journal of Physiology 515: 579–589, 1999. doi: 10.1111/j.1469-7793.1999.579ac.x.
- Jeukendrup AE, Jentjens RLPG. Oxidation of carbohydrate feedings during prolonged exercise: current thoughts, guidelines and directions for future research. Sports Medicine 29: 407–424, 2000.
- Jeukendrup AE. Carbohydrate and exercise performance: the role of multiple transportable carbohydrates. Curr Opin Clin Nutr Metab Care 13: 452–457, 2010. doi: 10.1097/MCO.0b013e328339de9f.
- Jentjens RLPG, Shaw C, Birtles T, Waring RH, Harding LK, Jeukendrup AE. Oxidation of combined ingestion of glucose and sucrose during exercise. Metabolism 54: 610–618, 2005. doi: 10.1016/j.metabol.2004.12.004.
- Jentjens RLPG, Underwood K, Achten J, Currell K, Mann CH, Jeukendrup AE. Exogenous carbohydrate oxidation rates are elevated after combined ingestion of glucose and fructose during exercise in the heat. J Appl Physiol 100: 807–816, 2006. doi: 10.1152/japplphysiol.00322.2005.
- Wallis GA, Rowlands DS, Shaw C, Jentjens RLPG, Jeukendrup AE. Oxidation of combined ingestion of maltodextrins and fructose during exercise. Med Sci Sports Exerc 37: 426–432, 2005. doi: 10.1249/01.MSS.0000155399.23358.82.
- Burke LM, Hawley JA, Wong SHS, Jeukendrup AE. Carbohydrates for training and competition. J Sports Sci 29: S17-27, 2011. doi: 10.1080/02640414.2011.585473.
- Thomas DT, Erdman KA, Burke LM. Nutrition and athletic performance. Med Sci Sports Exerc48: 543–568, 2016. doi: 10.1249/MSS.0000000000000852.
- Podlogar T, Bokal Š, Cirnski S, Wallis GA. Increased exogenous but unaltered endogenous carbohydrate oxidation with combined fructose-maltodextrin ingested at 120 g h−1 versus 90 g h−1 at different ratios. Eur J Appl Physiol 122: 2393–2401, 2022. doi: 10.1007/s00421-022-05019-w.
- Hearris MA, Pugh JN, Langan-Evans C, Mann SJ, Burke L, Stellingwerff T, Gonzalez JT, Morton JP. 13C-glucose-fructose labelling reveals comparable exogenous CHO oxidation during exercise when consuming 120 g/h in fluid, gel, jelly chew or co-ingestion. J Appl Physiol 132: 1394–1406, 2022. doi: 10.1152/japplphysiol.00091.2022.
- King AJ, O’Hara JP, Arjomandkhah NC, Rowe J, Morrison DJ, Preston T, King RFGJ. Liver and muscle glycogen oxidation and performance with dose variation of glucose–fructose ingestion during prolonged (3 h) exercise. Eur J Appl Physiol 119: 1157–1169, 2019. doi: 10.1007/s00421-019-04106-9.
- King AJ, O’Hara JP, Morrison DJ, Preston T, King RFGJ. Carbohydrate dose influences liver and muscle glycogen oxidation and performance during prolonged exercise. Physiol Rep 6: e13555, 2018. doi: 10.14814/phy2.13555.
- Baur DA, Schroer AB, Luden ND, Womack CJ, Smyth SA, Saunders MJ. Glucose-fructose enhances performance versus isocaloric, but not moderate, glucose. Med Sci Sports Exerc 46: 1778–1786, 2014. doi: 10.1249/MSS.0000000000000284.
- Smith JW, Zachwieja JJ, Péronnet F, Passe DH, Massicotte D, Lavoie C, Pascoe DD. Fuel selection and cycling endurance performance with ingestion of [13C]glucose: evidence for a carbohydrate dose response. J Appl Physiol 108: 1520–1529, 2010. doi: 10.1152/japplphysiol.91394.2008.
- Smith JW, Pascoe DD, Passe DH, Ruby BC, Stewart LK, Baker LB, Zachwieja JJ. Curvilinear dose-response relationship of carbohydrate (0-120 g·h-1) and performance. Med Sci Sports Exerc 45: 336–341, 2013. doi: 10.1249/MSS.0b013e31827205d1.
- Rowe JT, King RFGJ, King AJ, Morrison DJ, Preston T, Wilson OJ, O’Hara JP. Glucose and fructose hydrogel enhances running performance, exogenous carbohydrate oxidation, and gastrointestinal tolerance. Med Sci Sports Exerc 54: 129–140, 2022. doi: 10.1249/MSS.0000000000002764.
- Sutehall S, Muniz-Pardos B, Bosch AN, Galloway SD, Pitsiladis YP. The impact of sodium alginate hydrogel on exogenous glucose oxidation rate and gastrointestinal comfort in well-trained runners. Front Nutr 8: 1–12, 2022. doi: 10.3389/fnut.2021.810041.
Comments ()