Unveiling the Potential: New Research on Fat Oxidation at Higher Exercise Intensities
Exploring the impact of low-carbohydrate diets on substrate oxidation rates during exercise, Noakes and Volek highlight surprising increases in fat oxidation rates, even at high intensities, challenging traditional understandings of metabolic fuel use.
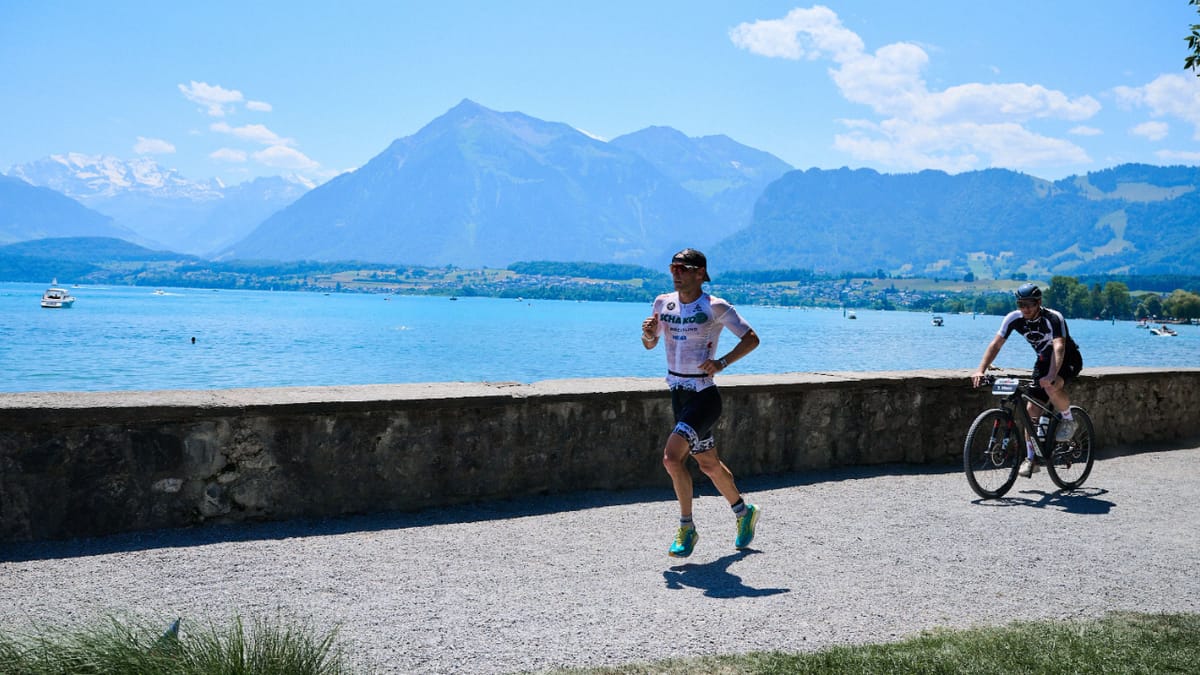
A thought-provoking review on low carbohydrate diets and substrate oxidation rates was recently published in the journal Frontiers in Physiology (10). The author list includes a couple of the big names in low carbohydrate research, Tim Noakes and Jeff Volek. In this blog, I will summarise some of the key messages and takeaways from the review.
The traditional model of exercise and substrate oxidation
During prolonged, endurance exercise, we primarily use two fuels to support our metabolism and keep us moving. We have carbohydrates, which are stored as glycogen in muscles and in the liver, and we have fat, stored in fat cells and in muscle. The rate at which we use carbohydrates and fats to support metabolism changes with things like exercise intensity and duration (14, 17), heat stress (6), and recent diet (2, 16). As our carbohydrate stores are relatively modest, we can become carbohydrate-depleted, and fatigued as a result, after exercise of sufficiently demanding intensity and duration. See training and fat metabolism for a refresher on this.
Noakes and colleagues start their review with a thorough overview of the relationship between exercise intensity and substrate oxidation rates; that is, the rate at which we are breaking down carbohydrates and fats to support energy metabolism, and therefore the exercise (10). Many, many studies have measured and reported this relationship (1, 3, 8, 15). Typically, we see an ‘inverted-U’ relationship between exercise intensity and fat oxidation rates, whereby fat oxidation rates increase with increasing intensity up to moderate workloads (below VT1), before decreasing at higher intensities. In contrast, as exercise intensity rises, the rate of carbohydrate oxidation steadily increases. See example curves from an individual athlete below (Figure 1).
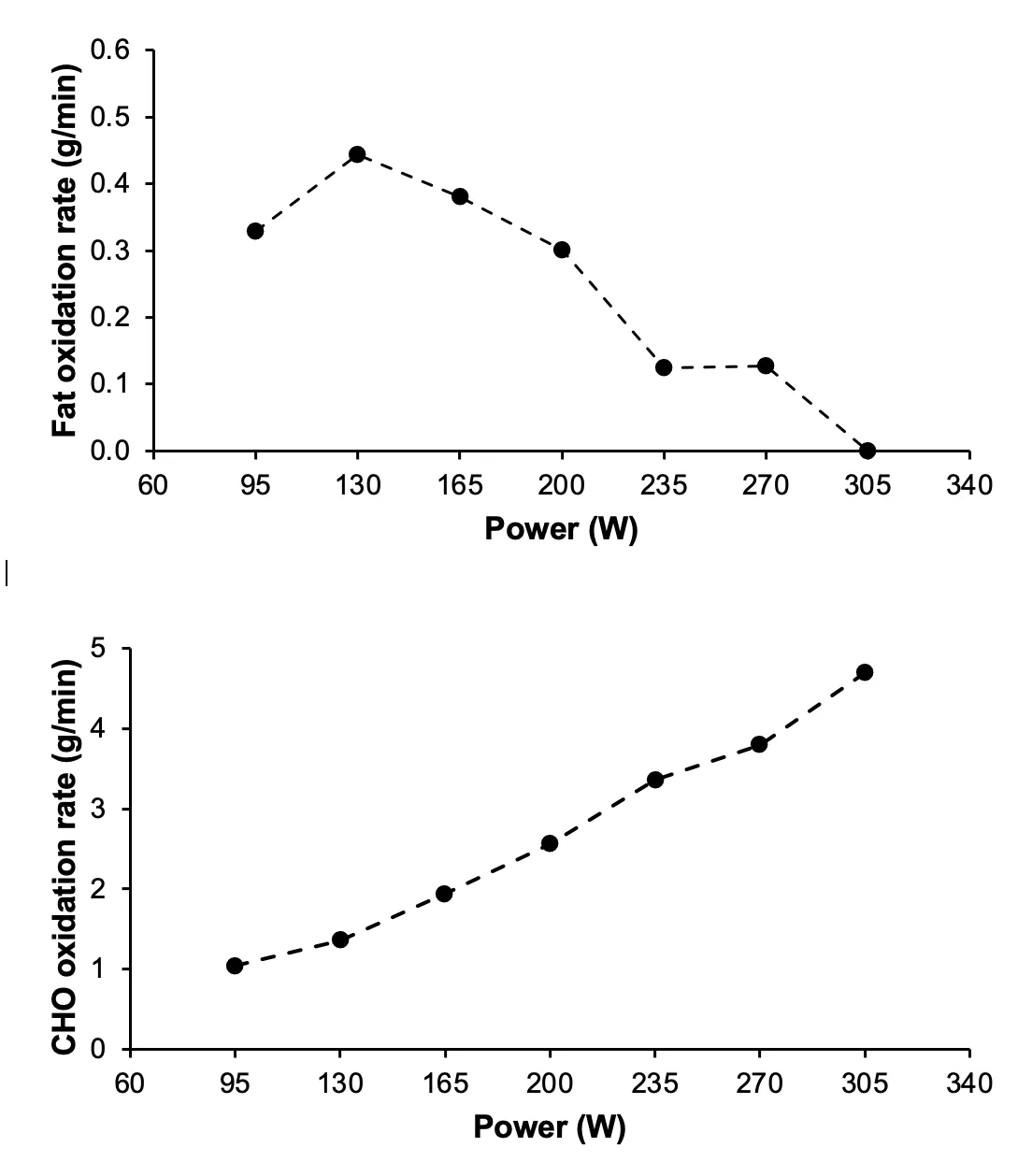
How these curves change in low-carbohydrate athletes
The description and curves above are typical of an athlete consuming a traditional, high-carbohydrate diet. In athletes consuming a low-carbohydrate diet, we see significant changes. We see higher fat oxidation rates across the board – higher peak rates, which generally occur at higher exercise intensities (4, 5, 9, 11, 13). See this effect in the example curves below (Figure 2); we have two athletes of similar fitness levels, but Athlete A (yours truly) consumes a low carbohydrate diet, and Athlete B a high carbohydrate diet.
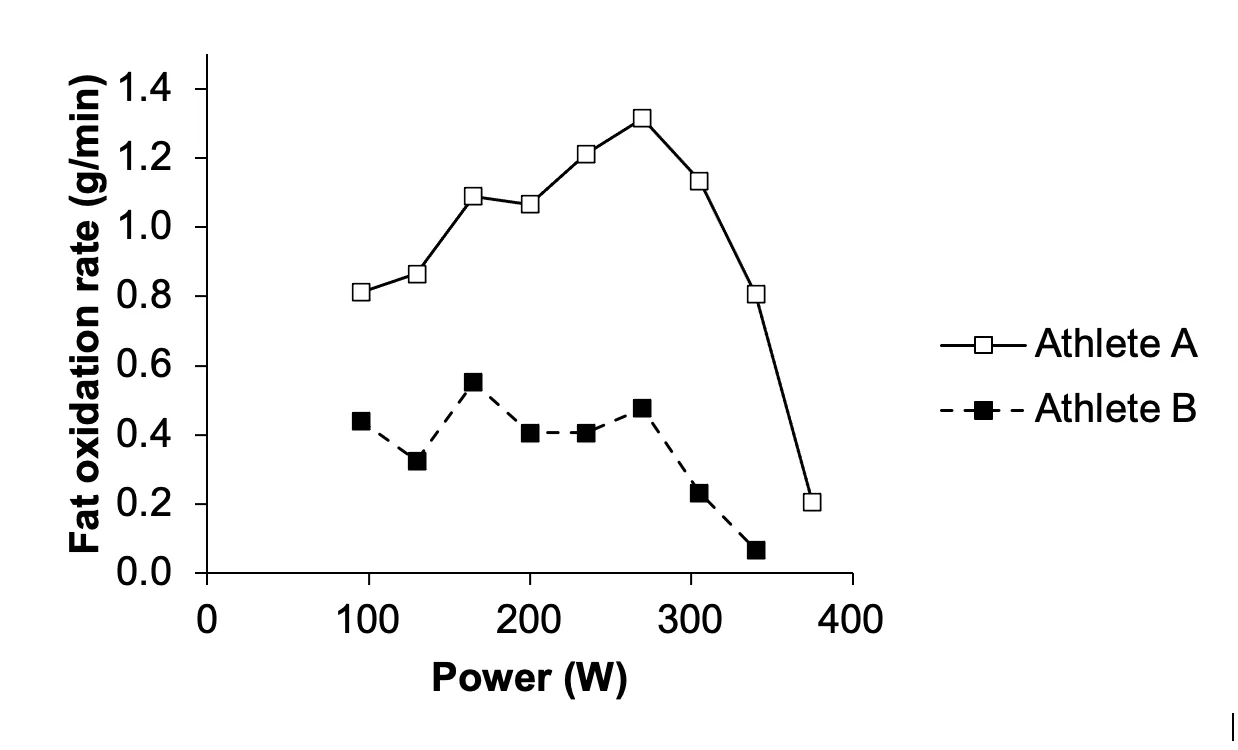
As pointed out by Noakes and colleagues, one of the interesting things about this upregulation of fat oxidation rates when ingesting a low carbohydrate diet is just how rapidly it occurs. Shifts in peak rates from ~0.5 to >1.0 g.min-1 can occur in as little as 5-7 days. We have seen this in research (5), but also in practice. That these changes occur so quickly tells us a little about the mechanisms at play. Specifically, these changes occur too quickly to be underpinned by fundamental, structural changes in the muscle itself, and instead are likely ‘unlocked’ by the more rapid changes in substrate availability and hormone concentrations. For example, insulin is a hormone that likely falls when ingesting a low carbohydrate diet, and insulin suppresses the breakdown of fats and promotes carbohydrate uptake by tissues. This suggestion, made eloquently in the paper by Noakes and colleagues, is substantiated by studies showing rises in fat oxidation over time during very prolonged exercise as glycogen falls (17), and by studies reporting elevated fat oxidation rates when training with depleted glycogen stores (7). Alongside these very rapid changes in fat oxidation rates, we should also acknowledge the possibility that some athletes may become slightly less efficient overall when ingesting a very low carbohydrate, or ketogenic, diet – meaning slightly more energy expenditure is required to run at a given speed, or cycle at a given power output (4, 13).
Fat as a fuel for high-intensity exercise
One of the striking findings in one of the newer studies of low carbohydrate diets and fuel oxidation – from Noakes’ group – is that fat can still provide a massive contribution to energy expenditure even at relatively high exercise intensities (85%V̇O2max, likely close to the second threshold) (11). Indeed, in that study, rates of >1.5 g.min-1 were observed, which is huge even at low exercise intensities. We traditionally think of carbohydrates as the dominant fuel source during high-intensity exercise – muscle glycogen is rapidly metabolised right where it is stored, is an oxygen-efficient fuel, and lactate production helps preserve NAD+ concentrations for proper downstream metabolic functioning. These results seem to suggest, with adaptation to a low carbohydrate diet, that these assumptions may need to be revisited. This study also reported unaffected high-intensity exercise performance, which again runs counter to the assumption that consuming a low carbohydrate diet will degrade glycogen-dependent high-intensity performance.
Interestingly, even in high-carbohydrate athletes, fat may provide a greater contribution to energy expenditure than our assumption of negligible rates at and above the second threshold. We use gas analysis to estimate fuel oxidation rates, and this method probably does systematically underestimate fat oxidation at high intensities (12). I won’t delve into the stoichiometry too deeply here, but I’ll just say that this underestimation likely occurs due to the extra carbon dioxide that is produced in the buffering of the metabolic acidosis that develops at high intensities, which pushes up RER and therefore decreases our estimate of fat oxidation.
Again, perhaps we need to reconsider the role that fat can play as a fuel source, even at high exercise intensities.
Summary and practical applications
Therefore, Noakes and colleagues have provided an excellent summary of the emerging low carbohydrate literature, and food for thought as we consider the energetic contribution that fat can make, particularly at high exercise intensities. If you are transitioning to a low carbohydrate, I definitely recommend you consider getting you exercise fat oxidation rates tested in an exercise physiology laboratory. The results might just surprise you.
References
- Achten J, Gleeson M, Jeukendrup AE. Determination of exercise intensity that elicits maximal fat oxidation. Med Sci Sport Exerc 34: 92–97, 2002.
- Achten J, Jeukendrup AE. The effect of pre-exercise carbohydrate feedings on the intensity that elicits maximal fat oxidation. J Sports Sci 21: 1017–1024, 2003. doi: 10.1080/02640410310001641403.
- Achten J, Jeukendrup AE. Maximal fat oxidation during exercise in trained men. Int J Sports Med 24: 603–608, 2003.
- Burke LM, Ross ML, Garvican-Lewis LA, Welvaert M, Heikura IA, Forbes SG, Mirtschin JG, Cato LE, Strobel N, Sharma AP, Hawley JA. Low carbohydrate, high fat diet impairs exercise economy and negates the performance benefit from intensified training in elite race walkers. J Physiol 595: 2785–2807, 2017. doi: 10.1113/JP273230.
- Burke LM, Whitfield J, Heikura IA, Ross MLR, Tee N, Forbes SF, Hall R, Mckay AKA, Wallett AM, Avish P. Adaptation to low carbohydrate high fat diet is rapid but impairs endurance exercise metabolism and performance despite enhanced glycogen availability. .
- Febbraio MA, Snow RJ, Stathis CG, Hargreaves M, Carey MF. Effect of heat stress on muscle energy metabolism during exercise. J Appl Physiol 77: 2827–2831, 1994.
- Marquet LA, Hausswirth C, Molle O, Hawley JA, Burke LM, Tiollier E, Brisswalter J. Periodization of carbohydrate intake: Short-term effect on performance. Nutrients 8: 755, 2016. doi: 10.3390/nu8120755.
- Maunder E, Plews DJ, Kilding AE. Contextualising maximal fat oxidation during exercise: Determinants and normative values. Front Physiol 9: 1–13, 2018. doi: 10.3389/fphys.2018.00599.
- McSwiney FT, Wardrop B, Hyde PN, Lafountain RA, Volek JS, Doyle L. Keto-adaptation enhances exercise performance and body composition responses to training in endurance athletes. Metabolism 81: 25–34, 2018. doi: 10.1016/j.metabol.2017.10.010.
- Noakes TD, Prins PJ, Volek JS, D’Agostino DP, Koutnik AP. Low carbohydrate high fat ketogenic diets on the exercise crossover point and glucose homeostasis. Front Physiol 14: 1150265, 2023. doi: 10.3389/fphys.2023.1150265.
- Prins PJ, Noakes TD, Buga A, D’Agostino DP, Volek JS, Buxton JD, Heckman K, Jones DW, Tobias NE, Grose HM, Jenkins AK, Jancay KT, Koutnik AP. Low and high carbohydrate isocaloric diets on performance, fat oxidation, glucose and cardiometabolic health in middle age males. Front Nutr 10: 1084021, 2023.
- Rowlands DS. Model for the behaviour of compartmental CO2 stores during incremental exercise. Eur J Appl Physiol 93: 555–568, 2005. doi: 10.1007/s00421-004-1217-z.
- Shaw DM, Merien F, Braakhuis A, Maunder E, Dulson DK. Effect of a ketogenic diet on submaximal exercise capacity and efficiency in runners. Med Sci Sports Exerc 51: 2135–2146, 2019.
- van Loon LJC, Greenhaff PL, Constantin-Teodosiu D, Saris WHM, Wagenmakers AJM. The effects of increasing exercise intensity on muscle fuel utilisation in humans. J Physiol 536: 295–304, 2001. doi: 10.1111/j.1469-7793.2001.00295.x.
- Venables MC, Achten J, Jeukendrup AE. Determinants of fat oxidation during exercise in healthy men and women: a cross-sectional study. J Appl Physiol 98: 160–167, 2005. doi: 10.1152/japplphysiol.00662.2003.
- Wallis GA, Rowlands DS, Shaw C, Jentjens RLPG, Jeukendrup AE. Oxidation of combined ingestion of maltodextrins and fructose during exercise. Med Sci Sports Exerc 37: 426–432, 2005. doi: 10.1249/01.MSS.0000155399.23358.82.
- Watt MJ, Heigenhauser GJF, Dyck DJ, Spriet LL. Intramuscular triacylglycerol, glycogen and acetyl group metabolism during 4 h of moderate exercise in man. J Physiol 541: 969–978, 2002. doi: 10.1113/jphysiol.2002.018820.
Comments ()